Multiple-beam interference: structural colours, iridescence …
Diffraction and interference
We first consider diffraction by a single slit and by a double slit. After passing a slit, the light beam widens. It widens the more, the narrower the slit is as compared to the wavelength.
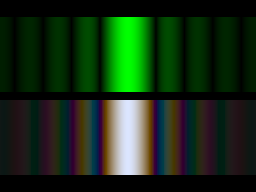 |
Diffraction on a single slit. Top: monochromatic light, bottom: white light. In the following we assume that the slits are very narrow and only look at what happens within the central bright region. The dark bands in the left picture would be far outside of the details shown in the following images.
|
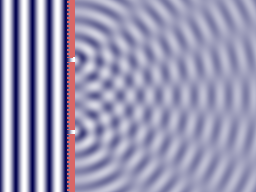 |
Diffraction by a double slit
A plane wave coming in from the left falls on an absorbing screen with two narrow slits. The outgoing waves on the right show alternating constructive and destructive interference. Double-click on the picture will switch on the animation, a single click switches it off again.
|
| Diffraction pattern of a double slit. Top: monochromatic light. This pattern corresponds to the animated picture above.
White light produces a coloured pattern (which in most experimental situations is rather dim and therefore the colours are not clearly seen).
|
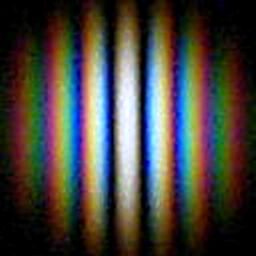 | A simple home experiment: Diffraction by a double hole. Two tiny holes were pierced with a sewing needle into an aluminium wrapping foil. Looking through these holes at a "point-like" light source, one can see a diffraction image similar to that shown here. For this image, the pierced foil has been fastened in front of the lens of a digital camera, the light source was the reflection of a halogen lamp in a small silvery glass ball (as used for christmas-tree decoration). The diameter of the holes was 0.07 mm, the centre-to-centre distance 0.3 mm.
|
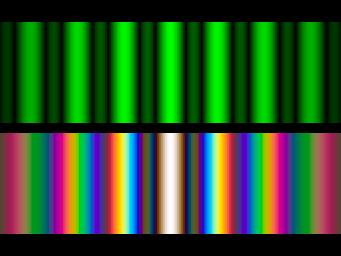 |
Three slits. Adding a third slit at the same distance, the intensity maxima are preserved. Between them there are now two dark bands and a faint secondary maximum. The pattern produced by white light shows more intense colours.
|
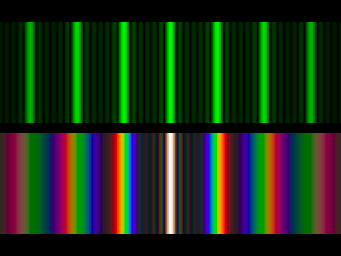 |
Seven equidistant slits: the bright "lines" are now separated by six dark bands and five faint secondary maxima.
The colours of the "first order" diffraction maximum on both sides of the central white beam approach saturated spectral colours. The short-wave (blue-violet) end of the third-order diffraction already overlaps the long-wave (red) end of the second order.
|
The more slits, the narrower are the main maxima and the weaker are the secondary maxima between them. The image below is for 15 slits.
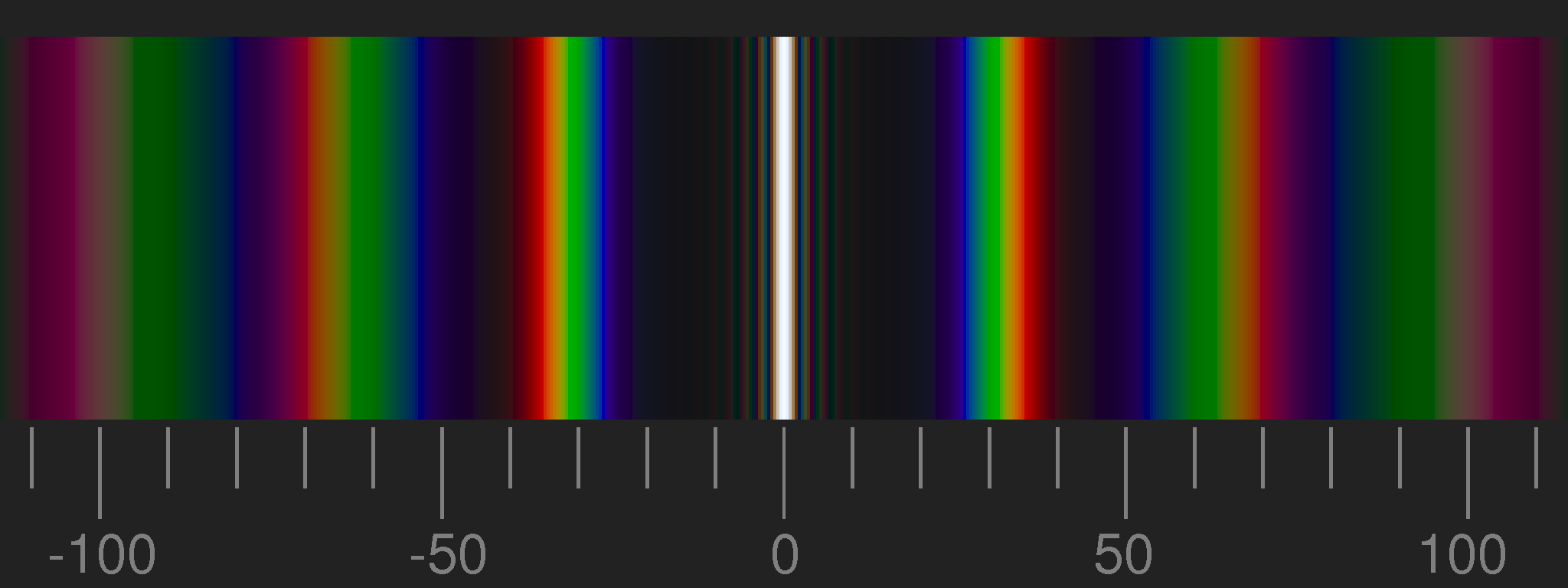
The scale gives the product of the lattice constant (= distance between centres of slits), measured in microns, and the deflection angle in degrees. It is only valid for small angles.
Optical diffraction gratings as used for spectrometers and spectroscopes are something like arrays of hundreds of slits. To make use of the obtainable high resolution, additional optical elements are necessary: a collimating lens to convert the divergent rays of the point source to a beam of parallel rays (plane waves), and a telescope to view the spectrum enlarged.
An array of reflecting stripes separated by mat areas has the same effect in reflected light as a transparent grating in transmission.
Examples
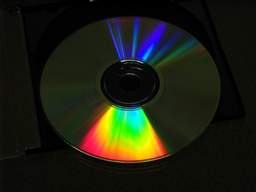 |
Everybody knows the vivid colours seen on compact discs. There is a mirror image showing no colour-splitting, but depending on the viewing angle, diffraction images of first or higher order can be seen. In case of small light sources, in first order almost pure spectral colours appear. |
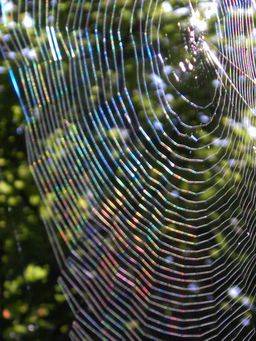 |
Under favourable conditions (dark background, but against the light) also spider webs may shine in vivid colours. For that, there are different possible reasons. In the picture shown, the main effect is due to the regular array of the glittering tiny sticky droplets on the capture threads. The web is shown approximately in natural size, intentionally slightly out of focus.
The pages "spider webs" present more images and give more detailed explanations. |
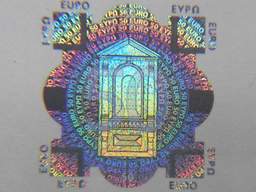 |
If instead of simple stripes a more involved pattern is produced, colourful images may result, as in the case of holograms. Best known examples probably are those seen on bank-notes.
|
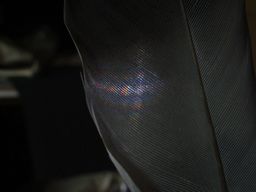 |
Birds' feathers consist of a hollow shaft with branches fused to it on opposite sides. The branches (barbs) carry barbules which in the feathers' vanes are so small, dense, and regularly ordered that they form a diffraction grating. The picture on the left shows the feather of a pigeon, illuminated by a white LED in the background. Below at the left an enlarged detail where the focussing was on the feather, at the right the same with focus on the light source in the background. |
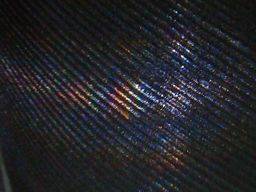 |
|
Iridescent colours
Only in exceptional cases the colours of natural objects are due to regular arrays of shiny areas separated by mat or dark areas. Quite frequent are interference colours due to more or less regularly stacked layers or other structures differing in their refractive index, so that they reflect light. Few transparent layers separated by small gaps produce vivid colours in reflected light.
Stacked layers
Stacked layers of alternating refractive indices are the simplest case to deal with.

Colours of a thin dielectric layer in reflected light before a dark background. These are the same colours which we know from
soap films. The scale gives the optical path difference of the two rays reflected on the upper and the lower surface in nanometers (nm).

Two transparent layers, separated by an air gap of the same optical thickness. The scale gives the optical distance between the front surfaces of the two layers. (Optical path length means the path length multiplied by the refractive index of the medium.)

Four transparent layers with refractive index n=1.55, separated by air gaps. The more layers, the narrower the maxima of reflected light and, correspondingly, the more saturated the colours.

Four transparent layers with refractive index 1.68 separated by three layers with index 1.55, on a thick substrate with index 1.55.
A typical feature of the colours seen on thin films and stacked thin transparent layers is iridescence, which means that the colour changes when the viewing angle is changed.
|
Reflectivity and colour of a stack of 16 double layers as a function of the viewing angle. Refractive indices are 1.45 and 1.6, optical thickness of a double layer is 300 nm. |
Details of the computation of the above pictures for stacked layers are given in the articles collected in the section "Computing Colours"; there are also sample codes for the images.
Examples
Mother-of-pearl: Seashells often show iridescent colours at the inner side. In particular, abalone shells (Haliotis sp.) may show vivid colours so that they may be used for costume jewellery.
Inner side of an abalone shell (Haliotis rufescens) at different observation angles.
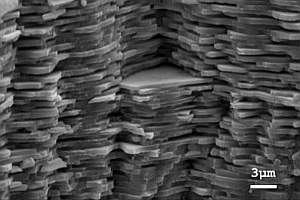 | |
Scanning electron micrograph of a transverse fracture of nacre. Photo: Fabian Heinemann, source: http://de.wikipedia.org. Click on the image for enlargement. |

Computed colours for a stack of layers of alternating index of refraction and optical thickness. The scale gives the optical thickness of one composite layer in nanometers (nm).
Mother-of-pearl
consists of thin aragonite tiles held together by layers of an organic polymer (conchiolin, chitin or other).
At each boundary between the crystals and the polymer, part of the incident light is reflected. Depending on their wavelength, the reflected waves may interfere constructively or destructively.
The layers between the tiles are thin. From a scanning electron micrograph of a transverse section of the shell of Haliotis glabra in a paper by T.L. Tan, D. Wong, and P. Lee, Optics Express 12 (2004), No. 20 p. 4847, (link) one can see that it is about one third of the thickness of the tiles or less.
The image above shows computed colours for a stack of 32 layers. Normal incidence is assumed for simplicity, the refractive indices are taken to be 1.685 (aragonite) and 1.57 (chitin), and the ratio of optical thicknesses 85:15.
As the colours depend sensitively on the parameters, exact agreement can not be expected. But a general property can be seen: vivid colours arise for thin layers; for a periodicity interval above 2 μm only pale greens and pinks are seen, which are the most common nacreous colours.
Reflectivity and colour of a stack of 1024 double layers, parameters as in the previous picture, optical thickness of a double layer 1480 nm. |
|
Shell of Haliotis asinina at different viewing angles
Meat: Iridescence may be observed sometimes also on meat, mostly reddish or pale green colours.
Pieces of ham. The left image is in natural size (approximately), the right one is magnified (4 x). The normal colour of the meat is partially masked by iridescence.
Striated muscles consist of fibers. The striae visible under the microscope reveal a regular layered structure, going along with periodic changes of the density and of the refractive index. Iridescence is only seen if the meat is cut more or less orthogonal to the muscle fibers, so that the striations are nearly parallel to the surface, like the layers in nacre.
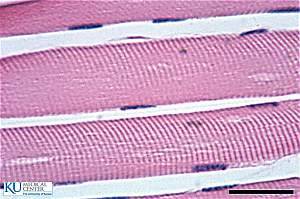 | |
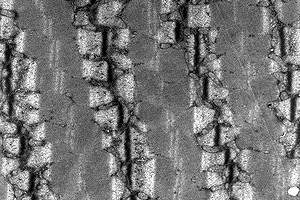 |
Striated muscles under the microscope (stained). The length of the bar in the lower right corner is 30 μm. © 2012 The University of Kansas Medical Center; authors: Milton Wolf & Marc Scarbrough.Source: The JayDoc HistoWeb | | Transmission elektron micrograph (TEM) of human striated muscle. Image width: 10 μm. Photo: Louisa Howard, Source: http://commons.wikimedia.org |
The black lines in the electron micrograph are the cross sections of the Z-discs which terminate the sarcomeres on both sides. The sarcomeres are, so to say, the periodic units of the muscle. The distance between Z-discs is approximately 2–2.5 μm at rest. Thus the index of refraction is periodically changing, and the length of one period is such that mainly greenish and pink iridescence is to be expected.
Labradorite, a feldspar mineral of the plagioclase series (NaAlSi3O8 to CaAl2Si2O8), is grey, transparent, and exhibits iridescent colours. This is due to the laminar structure of the crystals (unmixing lamellae of albite NaAlSi3O8 and anorthite CaAl2Si2O8). The two components have slightly different refractive indices. This leads to very small reflection of light at the boundaries, but the large number compensates the smallness. For some wavelength-regions, the waves reflected at the different boundaries interfere constructively, for others destructively. As in the case of soap bubbles and nacre, as shown above, the colour seen depends on the thicknesses of the laminae and on the viewing angle.
Left: A polished slab of labradorite from Madagascar, approximately natural size. Right: A cabochon of white labradorite ("rainbow moonstone") from Bihar, India. Length 23 mm.
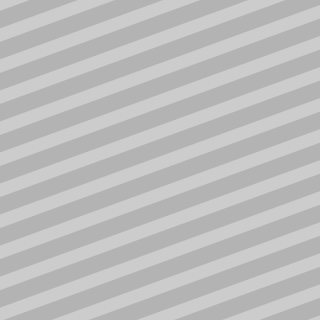
Left:
Composition of labradorite: alternating layers of slightly different refractivity.
Right: Result of a model computation of a stack of 1024 double layers (d1=80 nm, n1=1.518, d2=75 nm, n2=1.588). The colour depends strongly on the viewing angle.
Moonstone, another feldspar mineral, consists of orthoclase or microcline KAlSi3O8 with a small admixture of albite NaAlSi3O8. It shows an attractive pale bluish lustre called adularescence, from adularia, which is another name for moonstone. The sheen is caused by the layered structure of alternating potassium rich and sodium rich lamellae.
A small moonstone cabochon from Sri Lanka (length 8 mm approximately). The sheen comes from inside the stone and changes with illumination and viewing angle, as shown by the three pictures. Its colour, however, does not change perceptibly, it is always bluish white.
A strictly regular stack of alternating layers with different refractivity would produce strong iridescence, i.e. colours changing with the viewing angle, as demonstrated above in the case of labradorite. If, however, the orthoclase layers (which are much thicker than the albite lamellae, according to the composition of the stone) deviate randomly from their average thickness, the interference between the waves reflected from the different thin albite sheets is averaged out and the reflected intensities add up.
Transparent films which are much thinner than the wavelengths of light reflect short waves more than long ones (the reflectivity is then inversely proportional to the square of the wavelength). Therefore, with white illumination, the reflected light is bluish.
With increasing thickness, the reflection becomes white, then yellowish.
Left:
Schematic representation of moonstone composition. Right: Moonstone adularescence colours from a model calculation assuming irregular spacings of albite lamellae with thicknesses of about 50 nm or less (upper line) and 75 nm (lower line).
Three dimensional regular arrays
Opal is amorphous, i.e. noncrystalline silicon dioxide containing few percent of water. It is more or less milky and transparent. Precious opal shows variable colours inside. It consists of closely packed silica spheres of uniform diameter, so that the inhomogeneities form regular lattices, as can be seen in the REM pictures supplied by the Mineral Spectroscopy Server of the
California Institute of Technology: opal_gem, opal-beads. The spaces between the beads are filled with water or water-rich silica. Each of them scatters some light.
The scattering centres located in a plane behave very similar to a weakly reflecting plane, and three-dimensional lattice behaves like a stack of such planes. The situation is analogous to that of X-ray diffraction by crystals, only the scale is different. At certain angles, light with a certain wavelength is reflected strongest (according to Bragg's Law of diffraction). Changing the angle, the reflection maximum wavelength and thus the colour changes.
A small piece of raw opal from Ethiopia viewed at different angles. The width of each image is 15 mm.
Helical structures
Cholesteric liquid crystals consist of filiform molecules with a handedness. The molecules tend to align themselves parallel in planes, but from one plane to the next, the orientation changes by a small angle, leading to a screw-like structure.
As the polarizability of the long molecules is different in longitudinal and transverse directions, such an ordering produces a periodic change in the (anisotropic) index of refraction. Therefore, such a substance behaves similar to a stack of layers with alternating refraction index.
A sample of a cholesteric liquid crystal enclosed between acrylic glass and a black plastic backing. Such gadgets were popular in the 1970s as decorative objects or toys ("Space Fidgets"). The colour depends on the viewing angle. Touching the disc on the back side produces changing iridescence when the fluid elements are deformed. | |
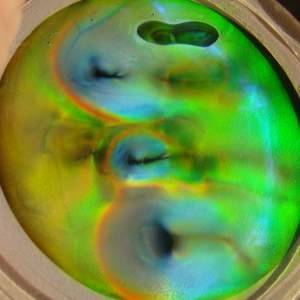 |
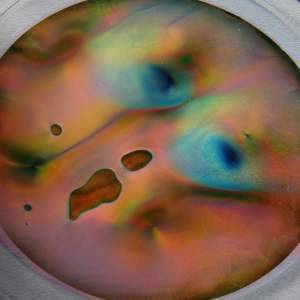 |
Compared with stacked layers, there is a remarkable difference: if the alignment corresponds, say, to a left-handed screw, the reflected light shows left-handed circular polarization (and vice versa). (If the rotation of the electric field is clockwise when the observer looks into the oncoming wave, the circular polarization is right-handed by definition, left-handed if the rotation is counter-clockwise.) A not too simple calculation shows that in the case of left-handed helical ordering, right-handed light is not reflected while incoming left-handed circularly polarized light is reflected as left-handed light. Reflection by ordinary mirrors would flip the handedness.
Left image: left-handed circularly polarized light, right image: right handed light. (The filtering was done with a linear pol-filter and a quarter wave plate in front of it.)
As a surprise, in both polarizations colours are seen.
The image to the right has been taken through a microscope. The width is 1 mm.
The liquid is not homogeneously stratified, but instead the orientation of the layers is constant only over small parts of the volume, which leads to a grainy, glinting appearance.
Presumably in the single domains the helicity is right handed, but the orientation of the domains in the volume is random. Light reflected by a deeper grain has to pass other obliquely oriented domains, and this introduces random phase shifts, thus reducing the degree of polarisation.
The large dark region in the left picture above, where the liquid film is very thin, represents near to ideal conditions as there all the layers are parallel to the boundaries.
|
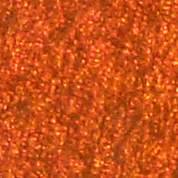 |
Iridescence
Iridescence means that the colour depends on the viewing angle.
In this respect, multilayer structures and helical liquid crystal structures behave similarly. With the latter, demonstration is easy.
First a preliminary remark. Iridescence may be distinguished from the gloss of the surface, if the iridescent structures in the volume are not exactly parallel to the surface. Where a dark object is mirrored at the surface, the light coming from deeper layers is seen (right image). The following photographs have been taken avoiding the bright reflections on the surface. |
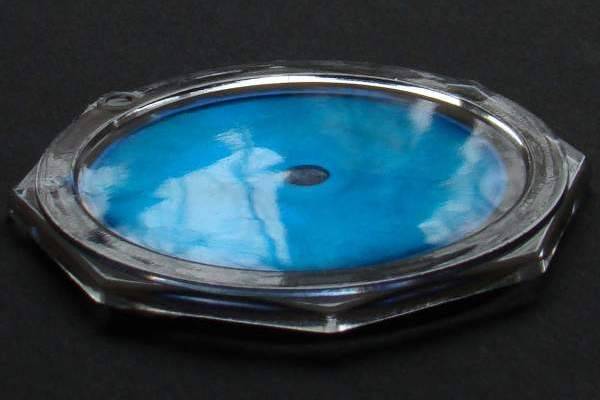 |
A sample of cholesteric liquid crystal under different viewing angles.
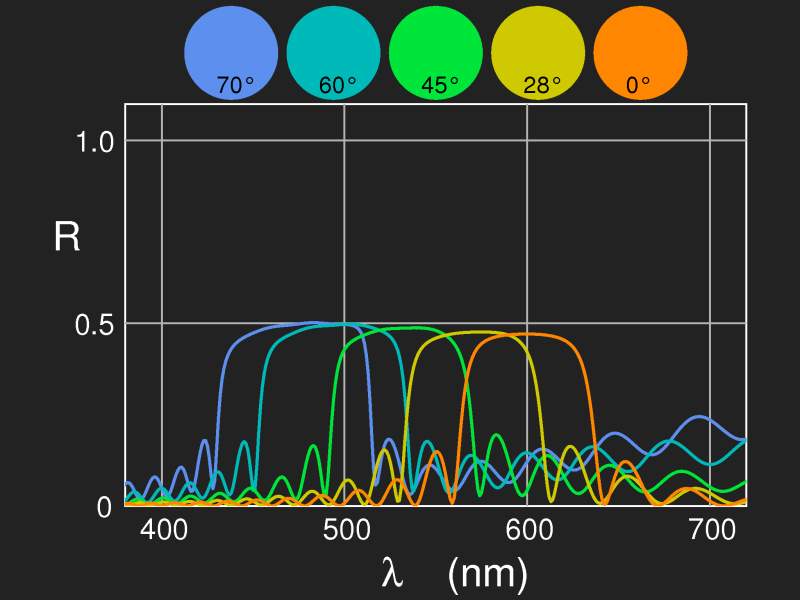 |
Reflectivity and colour of a cholesteric liquid crystal. Model computation with parameters nparallel = 1.60, northogonal = 1.45,
absorption coefficient κ = 0.002, pitch of the helical ordering H = 393.44 nm (corresponding to the optical periodicity interval of 300 nm), total height D = 4 μm.
|
Structural colours
Lustre and colours produced by special regular structures can often be found on birds and insects. Many examples are shown in the corresponding section of the chapter on colours of plants and animals.
The structures
Butterfly wings are covered with microscopic, partially overlapping scales. White, yellow, orange, red, brown, grey, or black colour of the scales can originate from their pigmentation, green pigments, however, are rare, and blue ones are very rare. In most cases, green and blue on butterflies' wings are structural colours; the other colours may occasionally be structural colours as well.
The image to the right shows pigmented and unpigmented scales from the wing of the Small Tortoiseshell
Aglais urticae. The image width is 0.8 mm.
|
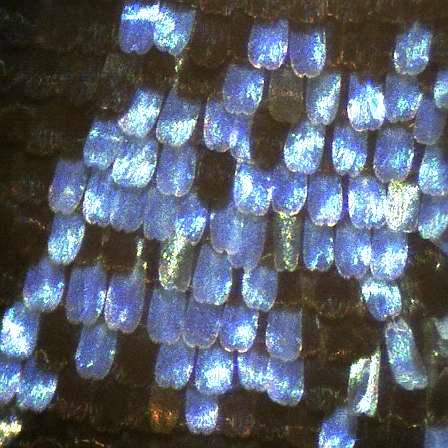 |
The scales of several species of butterflies and moths have been investigated by electron microscopy; pictures may be found in the web. Here is a link to an original research paper by R.O. Prum et al.(2006):
free download.
Comparatively simple are the scales of the magnificent, day-active moths of the genus Urania.
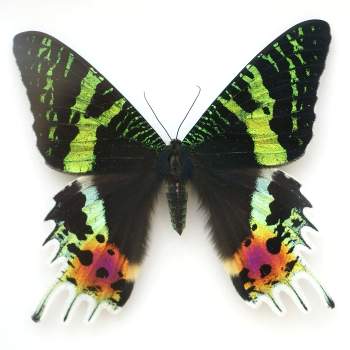 |
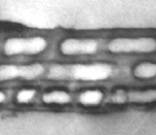 Cross section of an iridescent scale of Urania sp. (Source: pages of the School of Physics at The University of Exeter) |
Urania riphaeus from Madagaskar. (Source: FAAXAAL, public domain photos for the internet. Author: Kriss de Niort) | |
Four transparent layers of chitin separated by air filled gaps – this corresponds well to the model calculation image shown above.
Because of their gorgeous blue, the Morpho species have been investigated several times. Their iridescent scales are quite complex. They show thin parallel strips at their upper side. The electron microscope discloses the particular structure of these strips which are shown in the picture below in cross section.
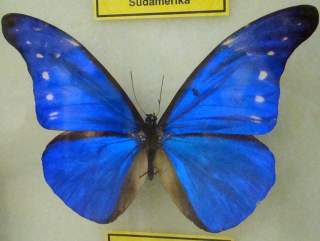 |
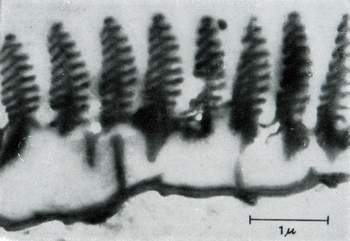 |
Morpho rhetenor cacica from South America. Specimen of the Insects' Museum in Wunstorf/Steinhude, wingspan ca. 15 cm.
| Part of a cross section of an iridescent scale of Morpho aega. Elektron microscope picture: W. Lippert. (From: Karl Gentil, Interferenzfarben ... , Zeiss Informationen 49, p. 80–81, about 1966) |
The image to the right shows an older photograph. Possibly the structure has been slightly deformed in the preparation of the slide.
Nowadays there are other pictures available, e.g. from the School of Physics of the University of Exeter.
Each of the strips consists of a regular arrangement of thin transparent chitinous layers. Altogether these form an array of reflecting surfaces, and the considerations are similar to those sketched in the case of opal. For a more thorough discussion, see this OPOD of the atmospheric optics site.
It is remarkable that the structural colours of many butterflies are hardly or not at all pavonine. How iridescence is suppressed has been investigated in a few species, among them the Blue Celastrina argiolus and the Green Hairstreak Callophrys rubi (Poladian et al. 2008).
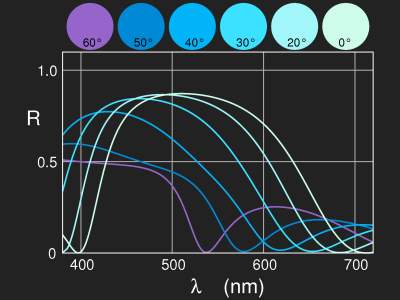 |
Reflectivity and colour of four chitin layers separated by three air-filled gaps. Optical thickness of each layer/gap is D = 127.5 nm. In view of the large changes of the colour it is marvelous how evolution has succeeded to produce structural colour without iridescence.
|
The blues, like the holly blue, Celastrina argiolus, use stacks of layers with air gaps in between, however, the layers are not continuous, but rather like an irregular network. Moreover, the upper surface is not smooth, but structured. This reduces the dependence of the colour on the viewing angle.
Right: Holly blue, Celastrina argiolus |
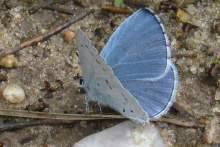 |
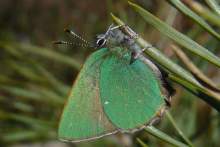 |
The scales of the green hairstreak, Callophrys rubi, contain regular cubic lattice structures (gyroid structures), see Michielsen et al. 2007, figures 5–7. On each scale, there are many small randomly oriented domains of few μm diameter only. By this, iridescence is averaged out and the scales look uniformly coloured (Poladian et al. 2008). |
See also the TEM images from the paper of Prum et al. (2006) Fig. 4, Fig. 5 .
Different mechanisms can be found in beetles to produce lustrous colours.
Tropical jewel beetles of the species Chrysochroa raja have been investigated by Noyes et al. (2007) who found multilayer structures with alternating index of refraction.
Left: Jewel beetle Belionota sp. from Nias/Indonesia, ca. 2.6 cm, Right: Jewel beetle Megaloxantha nishiyamai from Mindoro/Philippines, ca. 6.5 cm, Specimens from the Insects' Museum in Wunstorf/Steinhude.
The leaf-beetle Plateumaris sericea (L.) occurs in various hues. There is a simple sequence of layers at the surface: three layers with high refractive index, between them thicker layers with lower index. The layers between 50 and 120 nm thick in one special case (Hariyama et al. 2002).
In the following two examples, the same method of colour generation may be taken for granted, only the number of layers is not yet known.
Dead-nettle leaf beetle Chrysolina fastuosa |
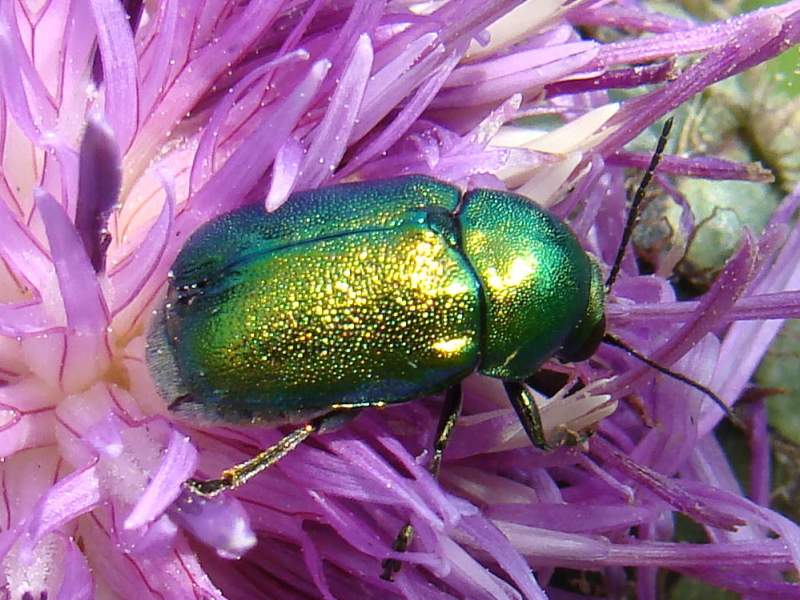 Cylindrical leaf beetle Cryptocephalus sericeus |
Chrysolina fastuosa has variable layer thicknesses, such that different parts glisten in different colours. Cryptocephalus sericeus has uniform layers, the colour changes seen are due to iridescence.
Many beetles (of the family Scarabaeidae) create their metallic colour in a different way. In layers the chitin molecules are aligned parallel to each other and to the surface. From one layer to the next the orientation angle changes by a small amount (like the steps in a corcscrew staircase), leading to a helical structure (similar to the ordering of molecules in a cholesteric liquid crystal, see above.). |
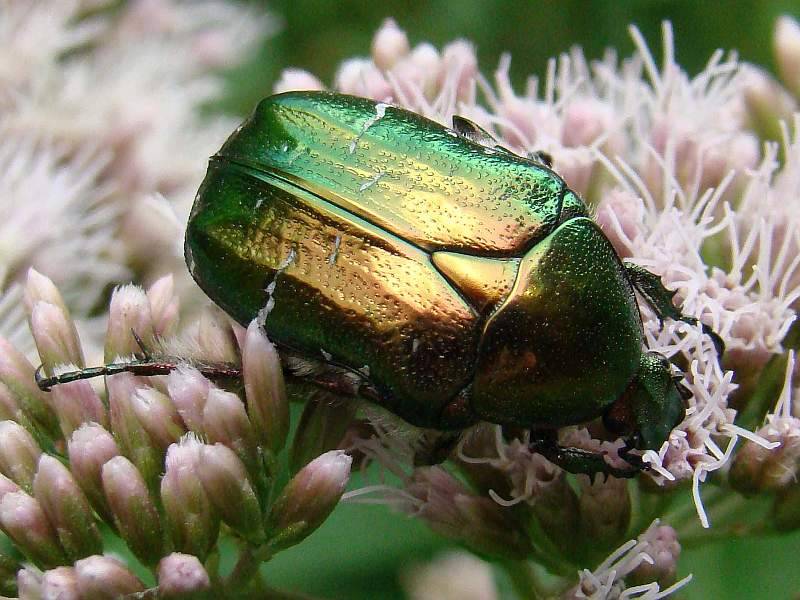
Rose chafer Cetonia aurata L. |
As the light reflected from such screw structures is circularly polarized, by analyzing the polarization of the reflected light, one can thus find out which of the two possibilities discussed is realized.
Cetonia aurata. Images taken through circular polarizing filters (from the glasses used for 3-d movies). In left circularly polarized light, the iridescent lustre is enhanced, while in right circular light the beetle is black.
Two corresponding images of a flower chafer, species Protaetia cuprea (= Protaetia metallica).
It is remarkable that the beetle looks metallic, iridescent colour changes are hardly noticeable. This is probably achieved by the microstructure of the surface, microscopic wrinkles, dimples or pimples. It would be interesting to see SEM images. |
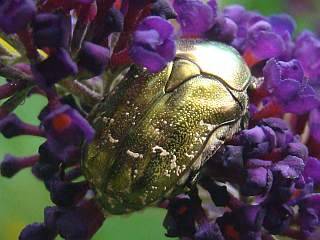 |
|
In beetles of the genus Plusiotis/Chrysina from South America, the helical molecular order in the cuticle has been discovered first, and species from this genus are still preferentially investigated.
Jewell et al. (2007) found microscopic dimples in the elytra of Plusiotis boucardi,
Sharma et al. (2009) the same in Chrysina gloriosa (synonym Plusiotis gloriosa).
"The fact that these jewel beetles reflect circular polarization was identified in the early 1900s by a Nobel Prize-winning physicist, A.A. Michelson, who hypothesized that the circular polarization might result from a 'screw structure' within the insect's cuticle, but he did not elaborate on it further."
(from Sharma et al. (2009):
"Structural Origin of Circularly Polarized Iridescence in Jeweled Beetles", see abstract).
For weevils, three-dimensional, opal-like structures have been identified as the mechanism producing sheeny colours. These regular structures are tiny and more or less randomly oriented in the scales on the surface of the insect. It is the same method of reducing iridescence which has been described for the green hairstreak above.
|
|
The weevil Phyllobius pomaceus Gyllenhal (Green Nettle Weevil, length 9 mm without antennae) is covered with greenish shimmering scales.
|
Elytron of Phyllobius pomaceus under the microscope. The width of the image is 0.5 mm (click for enlargement). |
|
|
A specimen of Polydrusus sp. (length 6 mm, found dead) under the microscope.
|
The elytron at higher resolution. The image width is 0.5 mm. |
Some exotic weevils use this mechanism for fancy colours, see e.g. the picture shown here together with an abstract of an interesting article in "Science".
An overview of all known sources of structural colour of beetles is given in the review article by Ainsley E. Seago et al., "Gold bugs and beyond: a review of
iridescence and structural colour
mechanisms in beetles (Coleoptera)", abstract with link to free download of the full text.
Birds:
Peacock's feathers are the best known example of birds' iridescence.
Left: The "eye" of a peacock's feather. On "mouseover", the viewing angle will change.
Right: greenish glittering barbules attached to the barbs. The barbules are flat and bending down and are sculptured by rows of shallow dimples (click to enlarge the picture).
Electron microscopy reveals the mechanism of colour generation:
In the cortex of the barbules, under a thin outer skin of keratin, several layers of melanin rods are arranged in a regular pattern. The rods are parallel to the surface and are held together by keratin. The cross section shows that they form a square lattice, with hollow spaces in the centres of the squares (Durrer 1962*, Zi et al. 2003).
Left:Transverse section through the cortex of a green barbule. The dark grey patches are the gaps between the rods. Right: Longitudinal section parallel to the surface.
Scanning electron micrographs from: Jian Zi, Xindi Yu, Yizhou Li, Xinhua Hu, Chun Xu, Xingjun Wang, Xiaohan Liu, and Rongtang Fu, Coloration strategies in peacock feathers (2003), PNAS. 100:12576-12578.
Copyright © 2003 National Academy of Sciences, USA; shown with permission.
The effect is similar to that of a stack of layers with alternating index of refraction, as discussed above for the case of precious opal. Different colours arise for different rod-rod distances (lattice constants).
Ducks and Pheasants
The black feathers on the head of the mallard drake (Anas platyrhynchos) are glistening green, or glistening blue if the angle between illumination and viewing is increased.
Glossy feathers of different species of ducks and pheasants have been investigared. Here also layers of melanin rods in the keratin matrix of the cortex have been found, but the arrangement of the rods is different from that in peacock feathers. The cross section shows a regular hexagonal, honeycomb-like lattice. (Rutschke 1966)*.
Hummingbirds produce their brillant colours with multilayer microstructures, stacked films made of melanin platelets containing air bubbles, effectively constituting alternating melanin and air layers.
Similar structures are employed by the Trogonidae. Layers of hollow melanin platelets, but also of hollow tubes of melanin. (A survey of the research on structural colours is given by Kinoshita 2008*.)
Reptiles
The green colour of reptiles arises from a combination of structural and pigmentary colour. On top there is a layer of xanthophores, i.e. cells containing yellow pigment which absorbs the blue light. Beneath is a layer of guanophores or iridophores, cells which contain nanocrystals of guanine suspended in the cytoplasm. If arranged irregularly, the guanine crystals scatter "Tyndall-like", the longer waves much less than the shorter ones. "Blue" is already absorbed, so what remains to be reflected is green. A bottom layer of melanophores provides a dark background.
If the guanine nanocrystals are arranged regularly, in addition to blue also other structural colours are possible, as in layered structures or opal.
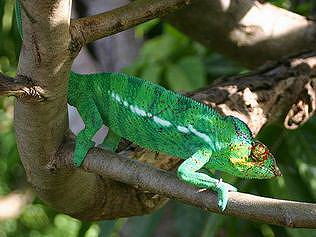 A chameleon of Madagascar (Photo: Brocken Inaglory ), source, license CC BY-SA 1.0 |
Chameleons are famous for their ability to change colour. In this, different mechanisms may be involved: the ability of the chromatophores to spread the pigment particles all over the cell or to concentrate them into a small lump, or, as published in 2015, tuning of the iridophores.
|
Teyssier, J. et al. (2015)
investigated the chameleon Furcifer pardalis from Madagascar. They found iridophores where the guanine nanocrystals form a regular face-centered cubic lattice. This is similar to the close-packing of spheres in opal, but the crystals do not touch. The orientation of the lattices is random, so that iridescence is averaged out. The colour is changed by changing the osmotic pressure, inducing swelling or shrinking of the iridophores by which the distance between neighbouring crystals is increased or reduced.
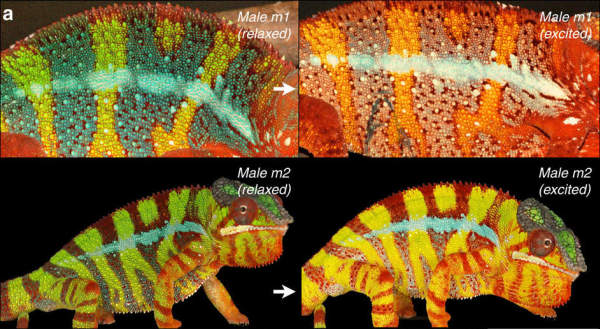
Colours of two males of the panther chameleon Furcifer pardalis. To the left in relaxed state, to the right excited by the presence of a rival male. Image taken from
doi:10.1038/ncomms7368, license
CC BY 4.0.
Photonic crystals
One-, two- or three-dimensional periodic arrays of refracting or absorbing elements in a matrix are called photonic crystals. (These arrays show band structures for light waves = photons which are similar to the energy bands of electrons = matter waves in crystals, hence the name.)
One-dimensional stacks of dielectric layers on glass are used as dielectric mirrors and colour filters. Two examples are shown in the images below.
Two stacks of eight composite layers, each layer consisting of a high-refraction and a low-refraction part of equal optical thickness, on a substrate with n=1.55. On the left hand side the refractive indices are 1.68 and 1.55, as found in the elytra of lustrous beetles by
Noyes et al. (2007), on the right hand side the indices are 2.3 and 1.38, corresponding to TiO
2 and MgF
2, substances which are used to produce dielectric mirrors.
Spectral reflectivity of the eight-layered stacks as described above, for 265 nm optical thickness of one composite layer. (Computations have been done with the transfer matrix method.)
Two- and three-dimensional photonic crystals are fields of research in nanotechnology and could perhaps soon find practical applications, while evolutionary nano-engineering has already produced wonderful examples in birds' feathers and butterflies' wings.
Diffraction vs. multilayer interference
Three ways of generating colour have been discussed here. The first is diffraction on grid-like surface structures, the second is interference of specular reflections on multilayer or three-dimensional lattice structures, without noticeable diffraction, and the third is reflection due to helically periodic variation of the refractive index. As in all cases the iridescent structures are sub-microscopic, one may wonder how to discriminate between them.
(Of course, also in the first case the colours are due to interference of the diffracted waves.)
To the right there is a gelatine-film replica of a diffraction grating with 13400 grooves per inch and the nacre shell of Haliotis asinina, in the light of an incandescent lamp.
| 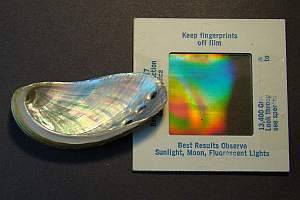 |
Let us compare some of the examples which I have already shown.
The first one is a hologram, which is something like a set of interwoven diffraction gratings.
Changing the angle of observation only a little bit, this results in considerable changes in perceived colour, as demonstrated by the following pictures:
The change in angle from the first one to the last is so small that the perspectivic distortion of the figure is hardly noticeable. Thus, even if we would not know that it is a hologram, we could infer that diffraction is the cause of the colours.
In addition, in this case there is (almost) always the zero order reflection without colours, as shown on the right-hand side. Colours are only seen if this specular reflection is avoided. Under diffuse illumination like the overcast sky no colours or only very faint ones can be seen. | |
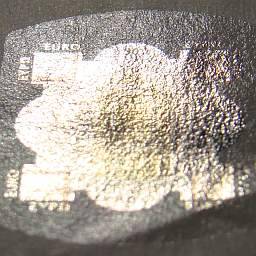 |
The next example is the mother-of-pearl inner side of an abalone shell. Again the colours change when the viewing angle is changed, but this time a large change of the angle makes only a small effect.
Inner side of the shell of a marine gastropod of the genus Haliotis at different observation angles: the angle was changed by 50° in order to produce significant changes in colour.
Clearly, diffraction does not contribute noticeably to the lustre, even if there might be a pattern of regular grooves at the surface.
The surface reflectivity is low, therefore any diffracted rays are weak while the multilayer interference yields reflections of much higher intensity.
Moreover, to see diffraction effects, one needs a light source with small angular extension. If there is only diffuse light as from the overcast sky, no diffraction effects are visible on the grating at any angle, while the nacre is shimmering in delicate pink and green. | |
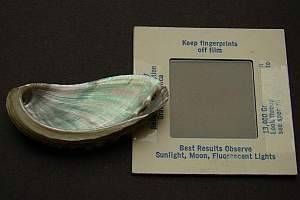 |
A compact disc illuminated by a small light source shows almost pure spectral colours (left), while diffuse light evokes very faint colours only or no colours at all (middle). If part of the diffuse light is shaded off, colours appear (right).
If they exist at all, natural objects which derive their colour from simple optical grating structures on their surface are extremely rare – I don't know any. Under the overcast sky they would loose their colour. While submicroscopic grooves on the surface of nacre may contribute to the lustre, I think that their effect has been grossly overestimated in some recent publications (1, 2).
Colours due to thin films, multilayer structures in particular, persist under diffuse light. The image to the right shows a polished specimen of labradorite from Madagascar, with a CD in the background which does not show any colour. |
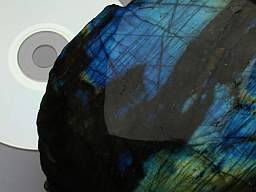 |
To summarize: iridescent colours which are constant over larger areas of the shimmering object, which do not depend strongly on the details of the illumination, and which change only little when you move your head (changing the viewing angle), are certainly not due to diffraction at the surface.
References
– | Heinz Durrer:
Schillerfarben beim Pfau (Pavo cristatus L.):
eine elektronenmikroskopische Untersuchung. Arbeiten aus der zoologischen Anstalt Basel, 21 Seiten, 1962
|
– | Shuichi Kinoshita: Structural Colors in the Realm of Nature. World Scientific 2008, ISBN-13 978-981-270-783-3
|
– |
E. Rutschke (1966): Die submikroskopische Struktur der Federn von schillernden Entenvögeln. Z. Zellforsch. 73, 432–443
|
– |
J. Teyssier, S.V. Saenko, D. van der Marel & M.C. Milinkovitch: Photonic crystals cause active colour change in chameleons. Nat. Commun. 6:6368 doi:10.1038/ncomms7368 (2015)
|
Back to the index page "the origins of colour"